MENU
|
FNCA 2002 WORKSHOP ON MUTATION BREEDING |
Application of mutation
breeding methods and
orientation of breeding in ornamental liliaceous plants
Keiichi Okazaki
Faculty of Agriculture, Niigata University,
Japan
|
|
|
|
Liliaceous species include many important ornamental
crops such as tulips, lilies, hyacinths, crocuses, narcissus and
alstroemeria. We have been selecting valuable varieties over a long
period of time and crops have been improved remarkably by using
different breeding techniques after the rediscovery of Mende's
Theory. In tulip, many cultivars have been developed through conventional
cross - breeding in Holland since 16th century. In the 1920, tulips
were introduced to the northern part of Japan, in Toyama and Niigata
prefectures, and Japanese original tulips have been developed. Recently
attempts have been made to apply mutation breeding for tulip.
In Japan, a large number of wild native Lilium species grow
and are also important as ornamentals. Lilium species became
domesticated and have been produced in the prefectures of Japan.
The exportation of lily bulbs was an important means of obtaining
foreign currency about one hundred years ago. During these years,
bulbs were dug in native habitats and exported. Through hybridization
among these native species, old cultivars of lilies had developed
about three hundred years ago and currently modern important cultivars,
'Asiatic Hybrids' and 'Oriental Hybrids', have
been developed. Cut-style pollination and embryo culture played
an important role in overcoming interspecific incompatibility and
recently, attempts have also been made to apply the mentor pollen
technique to overcome interspecific barriers.
In gladioli, bulbs are produced in Ibaraki prefecture. Mutation
breeding has been conducted at the Ibaraki Horticultural research
station. The culture of corms irradiated with gamma rays leads to
a high frequency of variants and production of solid mutants without
chimeras.
The objective of this paper is to briefly review the breeding of
liliaceous ornamental crops applied by using several techniques,
i.e. mutation, interspecific crosses and polyploidy. |
|
|
2. Bulb production
in Japan |
|
|
Tulips |
|
About one hundred and twenty
million bulbs are produced mainly in the prefectures of Toyama and
Niigata. These prefectures face the Sea of Japan where heavy snow
occurs in winter. Because snowfall prevents outbreak of aphid populations
in early spring, the transmission of virus diseases by aphids in these
areas is reduced. This is why these prefectures are suitable for the
production of bulbs. On the other hand, cut flowers are produced in
various prefectures such as Niigata, Saitama, Hyogo and Tokushima. |
|
Lilies |
|
About sixty million bulbs
are produced over an area of about 430 hectares in Japan. Nearly all
the production of lily bulbs is located in Kagoshima, Niigata, Hokkaido,
Toyama and Tottori prefectures. Kagoshima is the major producer of
L. longiflorum with about 20 million bulbs being grown over
180 ha. Most of the production area is located in the Okinoerabu Island,
which accounts for 93% of the production of L. longiflorum
in Japan. The major cultivar of L. longiflorum, 'Hinomoto',
which was selected from the natural habitat and propagated as ornamental
by scaling, accounts for above about 90% of the production in L.
longiflorum
'Asiatic Hybrids' has mainly been produced in the prefectures of Niigata
and Hokkaido and also the production of 'Oriental Hybrids' has increased
recently, although virus diseases and economic problems have limited
the production in each prefecture. Toyama prefecture, where L.
speciosum was the main cultivar, has experienced damage from the
development of Oriental Hybrids. However, some varieties of L.
speciosum are still being cultivated.
A large number of bulbs have been imported from Holland to Japan since
the end of the 1980 and about 200 million of lily bulbs has been exported
from Holland to Japan recently. In contrast, exports of bulbs from
Japan to Holland are almost inexistent. |
|
|
|
|
|
General aspects |
|
According to
the reviews of Broertjes and van Harten (1978) and van Harten (2002),
mutation breeding is suitable for the breeding of ornamentals mainly
because the new mutant varieties and the original ones have the same
genetic background except for the mutated gene. This implies that
the original and the new cultivars (mutants) may be produced under
the same culture conditions, while new cultivars obtained via cross
- breeding might require different growing regimes.
There are many polyploid varieties in ornamental plants such as tulips,
lilies, hyacinths, narcissus and alstroemeria. Especially, good triploid
varieties often appear. Since triploid varieties show aberrant meiosis,
which results in a high level of sterility and in the absence of seed
set, it is difficult to produce hybrids by using triploid parents.
In addition, asexual propagation of diploid cultivars over a long
period of time also leads to seed sterility. In such cases, mutation
breeding is the best method to generate variation.
The third reason why ornamentals are suitable for mutation breeding
is that economically important traits like flower characteristics
can be easily monitored after mutagenic treatment. In addition, since
many cultivars are heterozygous, they display a comparatively high
mutation frequency. |
|
Tulip |
|
In tulip, extensive studies on mutation breeding were conducted
in Japan, especially in Toyama prefecture (Myodo 1942, Nezu 1962,
1963a,b, 1964, 1965, Nezu and Obata 1964a, b). The effect of acute
and chronic irradiation on the growth of tulip plants was examined
(Nezu and Obata 1964a). The bulbs were irradiated in two ways; namely,
using a total amount of 10 Gy with dose rates ranging from 0.1 Gy
to 2 Gy /h, and by chronic 0.068 Gy/h irradiation the total amount
ranged from 20 to 80 Gy (Fig.1, Table 1). |
|
Table 1. |
|
Dose rate effect of gamma irradiation
on bulbil yield in two varieties exposed to 10Gy total dose
(Index, control 100) (Nezu and Obata 1964a). |
|
|
Variety |
Dose
rate
(Gy/h) |
Number
of bulbil |
Weight
of bulbil |
Total |
Large
a |
Small |
Total |
Large |
Small |
Diana
(diploid) |
0.1 |
105 |
90 |
111 |
91 |
73 |
135 |
0.18 |
124 |
83 |
141 |
95 |
63 |
172 |
0.5 |
138 |
84 |
159 |
83 |
50 |
164 |
1 |
110 |
9 |
163 |
49 |
5 |
156 |
2 |
124 |
7 |
171 |
41 |
4 |
131 |
Keizers
Kroon
(Triploid) |
0.1 |
104 |
127 |
88 |
94 |
93 |
96 |
0.18 |
111 |
129 |
99 |
97 |
94 |
106 |
0.5 |
91 |
131 |
64 |
82 |
83 |
80 |
1 |
110 |
126 |
99 |
71 |
67 |
84 |
2 |
110 |
87 |
126 |
63 |
49 |
112 |
|
a: |
|
Large bulbils measure 8cm in circumference
and above, while small bulbils measure less than 8cm in circumference. |
|
|
Ten Gy in the case of acute
irradiation with a dose rate of 2 Gy/h inhibited the elongation of
the flower stalk (Fig. 1). To obtain the same effect as that of acute
irradiation, chronic irradiation of 80 Gy with a dose rate of 0.068
Gy/h was necessary. There were some differences in the radiosensitivity
between diploid and triploid cultivars. The diploid cultivars were
more susceptible than the triploid ones to acute irradiation, while
there was little difference in radiosensitivity in the case of chronic
irradiation between diploid and triploid cultivars.
The total dose of 10 Gy in the case of acute irradiation of 1 Gy/h
caused a 50% reduction of bulb yield, while the number of bulbils
was not appreciably affected and even increase (Table 1).
These results suggest that the optimum dose is about 5Gy with a rate
of 1 Gy/h, 10 Gy with a rate of 0.5 Gy/h or 20 Gy with a rate of 0.05
Gy/h. |
|
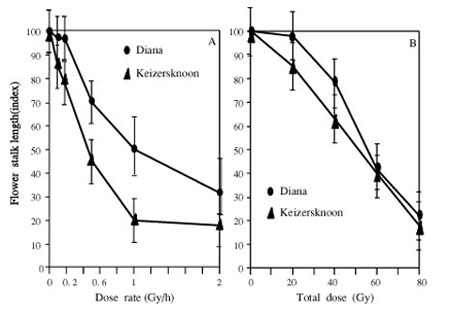 |
|
Fig. 1. |
|
Effects of acute (A) and chronic
(B) irradiation on the growth of the flower stalk, exposed to
a total dose of 10Gy and dose rate of 0.068Gy/h, respectively
(Nezu and Obata 1964a). |
|
|
The problem is when to irradiate. To answer this question, we need
to consider the life cycle of the tulip bulb. When the floral meristem
was irradiated at earlier developmental stage after harvest in June,
a larger mutated spot appeared on the perianth in the next spring.
In summer, the flower meristem gradually grows up in the bulb and
the morphogenesis is completed in September. When bulbs were irradiated
in September, small splashed spots were consequently observed on
the perianth in the next spring (Nezu 1963). The changes in the
flower color observed in the first generation after gamma irradiation
were due to the presence of mericlinal chimera and this mutated
sector in the perianth was not transferred to the next generation.
A larger mutated area spreading over 2 perianths was observed after
the second generation.
It is important that the mutation of gene, which controls flower
color, occurs in the vegetative cell line forming the next new bulb.
When the secondary bulblets are formed in the bulb in autumn, the
apices in the secondary bulblet are suitable for irradiation and
the selection should be started after two or three generations to
give a mutated cell the opportunity to express itself in the complete
mutant.
Flower color mutation, which can be easily monitored in the subsequent
generation after mutagenic treatment, was promoted in Toyama prefecture,
but new mutant varieties have not been developed in Japan, yet.
Flower color mutation, especially in the top tulip varieties, exerts
a considerable impact to customers and growers. However, the ranking
of top varieties often changes and it is difficult to anticipate
which will be the leading variety in the future. In tulip, since
it takes about 15 years to propagate a new mutant practically, the
application of mutation breeding is delayed |
|
|
4. |
Interspecific hybridization |
|
|
L. formosanum can be easily propagated through
seeds and has the ability to flower within one year after the sowing
of seeds. Nishimura, in Nagano, started crossing L. formosanum
with L. longiflorum in about 1928 and then developed L.
x formolongi which combines the characteristics of flowering
within one year after of sowing the seeds with the presence of broad
leaves like in L. longiflorum. To obtain the configuration
of L. longiflorum, L. x formolongi has been
backcrossed to L. longiflorum in the recent varieties. As
L. x formolongi is propagated by seeds, viral infection
does not occur. The other advantage is that cut flowers of L.
x formolongi can be produced from July to November, which
it is difficult to produce the cut flowers of L. longiflorum
in these months. It has been estimated that about 15 million cut
flowers of L. x formolongi are being produced. The
trend of lily breeding and production in Japan was reviewed by Okazaki
(1996).
Two major horticultural lily groups are referred to as 'Asiatic
Hybrids' and 'Oriental Hybrids'. The former are derived from species
such as Lilium maculatum, L. dauricum, L. lancifolium,
L. maximowiczii, etc. The latter are derived from such species
as L. speciosum, L. auratum, etc. Since these hybrid
groups were obtained from crosses between closely related species,
sexual reproduction barriers between interspecific crosses were
relatively low and hybrids could be easily obtained through conventional
cross - breeding.
Embryo culture has been found to be useful to overcome interspecific
incompatibility caused by insufficient endosperm formation in lilies
(Nakajima 1940, Emsweller et al. 1962, North and Wills 1969, Ronald
and Ascher 1976). Recently attempts have been made to develop crosses
between distantly related species to obtain newer interspecific
hybrids (Asano and Myodo 1977a,b, Van Tuly 1991, Okazaki et al.
1992, 1994). Cut-style pollination is suitable for overcoming interspecific
pre-zygotic barriers which inhibit the elongation of pollen tubes
in the style.
Asano and Myodo (1977) showed that MS medium is suitable for the
culture of immature embryos when it is adjusted to pH 5.0 and supplemented
with 20 - 40 g/l of10-4 - 10-2 mg/l NAA. Okazaki et al. (1994) adjusted
the optimum sucrose concentration to 6%. The techniques of nurse
culture (Asano 1980) and ovary slice and ovule culture (Hayashi
et al 1986, Kanoh et al. 1988, Van Tuyl et al. 1991) are also useful
for overcoming interspecific barriers. |
|
|
5. |
Mentor pollen technique |
|
|
Kunishige and Hirata (1972) and van Tuyl et al.
(1982) applied the mentor pollen technique to overcome interspecific
pre-zygotic barriers in Lilium. The mentor effect is due to pollination
with mixtures of compatible (mentor) and incompatible pollen. To
avoid prior fertilization by the compatible mentor pollen, mentor
pollen is killed by a high radiation dose. The irradiated pollen
is unable to induce the development of fertilized eggs, but is capable
of elongating the pollen tube. In lily, when pollen was irradiated
for ten hours with dose rate ranging from 3 to 20 Gy/h, the occurrence
of seed set gradually decreased when the dose increased (Table 2)
and the optimum dose was consider to be 100Gy to obtain pollen with
a mentor effect. Similarly, Van Tuyl et al. (1982) showed that lily
pollen irradiated at 100 Gy was much more effective in stimulating
the fruit set than pollen exposed to a dose of 250 Gy. By applying
the mentor pollen technique in a cross of L. longiflorum
x L. 'Asiatic Hybrid', in which the seed set is absent in
a normal cross, interspecific hybrids were obtained by using irradiated
L. longflorum pollen as a mentor (Van Tuyl et al, 1982).
Mentor pollen techniques have been successfully applied to overcome
incompatibility as reported by Stettler (1968) in Populus, Dayton
(1974) in apple, Den Nijs & Oost (1980) in Cucumis, Visser
(1981) in apple and pear and Ureshino et al. (2000) in azaleas.
Recently, Visser (1981) has observed that the seed set after pollination
with compatible pollen was considerably improved by applying the
pollen twice, with an interval of 1- 2 days. The pollen in the first
application was referred to as 'pioneer pollen' as it
appeared to promote the activity of the pollen in the second application.
It remains to be determined whether there is a difference in the
mentor effect between the mentor and pioneer pollen techniques. |
|
|
Table 2. |
|
Seed set of Lilium 'Asiatic
Hybrid' cv. 'Connecticut King' crossed with control
and irradiated pollen of 'Kiyobubeni' |
|
|
Cross-combination |
Dose
(Gy) |
No. of
pollinated
flowers |
No. of capsules obtained |
No. of seeds |
No. of seeds per
capsule |
No. of abnormal
seeds |
Connecticut King
Kiyobubeni |
0 |
5 |
5 |
536 |
107.2 |
31 |
30 |
5 |
5 |
165 |
35.0 |
69 |
50 |
5 |
5 |
60 |
12.0 |
28 |
100 |
5 |
5 |
1 |
0.2 |
1 |
200 |
5 |
5 |
0 |
0 |
0 |
|
|
|
|
|
In ornamental plants, a large number of polyploid
cultivars have been developed, because they display horticulturally
desirable characteristics such as good bulb production and larger
flowers. In Easter lily, Lilium longiflorum, successful induction
of polypoids by colchicine was reported (Emsweller and Lumsden,
1943; Emsweller ,1949; Emsweller and Uhring, 1960). These
reports indicated that tetraploid Easter lily had larger but fewer
flowers and thicker leaves and bloomed later than the diploid form.
Characters such as reduced flower number were improved in the progeny
obtained from crosses among tetraploid individuals. In addition,
the range of variation occurring in the tetraploid seedlings was
wider than that in any diploid populations. Thus breeding of Easter
lily on the tetraploid level is considered to be useful, although
tetraploid commercial varieties of Easter lily have not yet been
developed.
In the case of Lilium 'Asiatic Hybrid', commercial tetraploid
varieties have been distributed to markets recently. However, it
remains to be determined what kinds of changes occur with polyploidization.
The tetraploid sport, which was derived from natural polyploidization,
was compared with the original diploid clones under natural conditions
and early forcing culture (Okazaki et al. 2002). It was observed
that mean stomatal length of the original clone and its sport which
was 80μm and 114μm, respectively, was significantly
different by t-test. The increase of the stomatal size in the sport
appeared to be due to tetraploidization. Actually, McRae (1987)
observed the size of stomata in 40 varieties of L. 'Asiatic
Hybrid' and its wild relatives, and demonstrated that the length
of the stomatal guard cells was 67 to 83μm in the diploid
and 100 to 150μm in the tetraploid clones. The size
of the pollen of the sport which was 79.7 ±,0.43μm
(ave. ±,SD) did not differ from that of the diploid clone.
McRae (1987) reported that the pollen size ranged from 67 to 100μm
in diploid lilies and 90 - 150μm in tetraploid ones.
In general, a plant is composed of three cell layers, L1 -
L3, and reproductive tissue is produced in the L2 layer (Raven et
al. 1999). The pollen size of the sport showed that the L2 layer
was diploid, and, as mentioned above, the L1 layer corresponding
to the epidermis of the sport of 'Kiyotsubeni' was tetraploid.
This sport consisted of periclinal chimeras with a 4n chromosome
number in the L1 layer and 2n in the L2 layer.
Morphological and physiological changes associated with polyploidization
were compared between the diploid clones and the tetraploid ones
under natural conditions and early forcing culture. The morphological
differences between the diploid and tetraploid clones showed a similar
tendency under the natural and forcing conditions. Compared with
the diploid clones, the tetraploid clones displayed a reduction
in the number of flowers, a shorter stem and broader leaves. Under
early forcing culture at a low light intensity, the stems of the
tetraploid clones were softer than those of the diploid ones. Leaf
scorch frequently occurred in the diploid clones (72.2%), while
the rate of leaf scorch was 2.3% in the tetraploid clones. It is
concluded that breeding of Lilium 'Asiatic Hybrid'
at the tetraploid level is highly suitable.
This sport, which consisted of periclinal chimeras with a 4n chromosome
number in the L1 layer and 2n in the L2 layer, propagated without
reverting to the diploid form for a long period of time. A periclinal
chimera with different polyploidy levels between layers is always
stable in plants (Tilney-Bassett 1986). |
|
|
7. |
Chimeras in mutations and polyploids |
|
|
In mutation breeding, the mutated sector should
to be transformed into a solid mutant or a periclinal chimera. The
flower color depends mainly on the genetic constitution of the L-1
layer of the flower petals and, in chrysanthemum, a mutated flower
color was often obtained in different genetic constitutions between
L1 and L2 layers (Shibata and Kawata 1986, Shibata et al.1998).
In such periclinal mutants, it is important that periclinal chimeras
are maintained as long as vegetative propagation takes place. In
general, mutated cell lineage is stable in the periclinal chimeras,
but not in the mericlinal chimeras. This situation is the same as
that of chimeric polyploidy.
Chimeric condition will be terminated when chimeric plants propagate
by seeds. For instance, when a mutation exists in L1 and is lacking
in L2 and L3, this mutation cannot be transmitted to the next generation
via sexual reproduction, because of the formation of gametes in
the L2 layer. Actually, when the above-mentioned chimeric plant,
consisting of periclinal chimeras with a 4n chromosome number in
the L1 layer and 2n in the L2 layer, was crossed with a diploid
parent, most of the progenies were diploid and the tetraploid level
in L1 layer was not transmitted to the next generation (Okazaki
unpublished), indicating that the L1 layer does not contribute to
the production of gametes.
It should be noted that the radiosensitivity of cells in various
layers vary in the periclinal chimeras in which the layers differ
in the ploidy level (van Harten, 2002). Tetraploid cells are likely
to be more resistant to irradiation than diploid cells, because
doubled chromosomes in tetraploid cells compensate for chromosomal
damages associated with irradiation. As a result of such effects,
diploid cell layers may be replaced by tetraploid cell layers when
shoot apices of periclinal chimeras in which the layers differ in
ploidy level are irradiated. Actually some authors have demonstrated
that radiation treatment can be used to transfer a mutation that
is present only in the cells of one layer, to another layer (van
Harten, 2002). |
|
|
8. |
Application of in vitro culture in
mutation breeding |
|
|
In gladioli, when the meristems were cut from the
corm irradiated with gamma rays and then cultured in vitro, the
plantlets were regenerated via embryogenesis and showed a high frequency
of variants without chimeras (Kasumi et al. 2001). The total dose
of 100 - 200 Gy at 10Gy/hr caused a 50% reduction in the rate of
callus formation and somatic embryogenesis. The pink flower color
in the original cultivars 'Traveler' changed to a deeper
or paler pink colors in the mutated plants and a large number of
plants with a deep flower color were observed compared with those
with a pale flower color.
This propagation technique in combination with gamma irradiation
is an effective way of producing solid mutants, since the regenerated
plantlets of gladioli develop from one original cell via embryogenesis,
as described in chrysanthemum (Broertjes and Roest 1976, Nagatomi
et al. 1998). |
|
|
References
|
|
Asano, Y. and H. Myodo
(1977a) Studies on crosses between distantly related species of lilies.
I. For the intrastylar pollination technique. J. Japan. Soc. Hort.
Sci. 46:59-65. |
|
Asano, Y and
H. Myodo (1977b) Studies on crosses between distantly related species
of lilies II. The culture of immature hybrid embryos. J. Japan. Soc.
Hort. Sci. 46:267-273. |
|
Asano, Y (1980) Studies
on crosses between distantly related species of lilies IV. The culture
of immature hybrid embryos 0.3Å`0.4mm long. J. Japan. Soc. Hort.
Sci. 49:114-118. |
|
Broertjes, C. and S. Roest
(1976) Mutation breeding of Chrysanthemum morifolium Ram. using
in vivo and in vitro adventitious bud technique. Euphytica 25:11-19. |
|
Broertjes,
C. and A.M.van Harten (1978) Application of mutation breeding methods
in the improvement of vegetatively propagated crops. Elsevier, Amsterdam. |
|
Dayton, D.F. (1974) Overcoming
self-incompatibility in plants with killed compatible pollen. J. Am.
Soc. Hortic. Sci. 99:190-192. |
|
Den Nijs A.P.M. and E.H.
Oost (1980) Effects of mentor pollen on pollen-pistil incongruities
among species of Cucumis. Euphytica 29:267-271. |
|
Emsweller, S.L., S. Asen
and J. Uhring (1962) Lilium speciosum x Lilium auratum.
Lily Yb., North Am. Lily Soc. 15:7-15. |
|
Emsweller, S. L. and D.
V. Lumsden (1943) Polyploidy in the Easter lily. Proc. Am. Soc. Hort.
Sci. 42:593-596. |
|
Emsweller, S. L. (1949)
Colchicine induced polyploidy in Lilium longiflorum. Am. J.
Bot. 36, 135-144. |
|
Emsweller,
S. L.and J. Uhring (1960) Breeding Lilium longiflorum at the
tetraploid level. Proc. Am. Soc. Hort. Sci. 75, 711-719. |
|
Hayashi, M., K. Kanoh and
Y. Serizawa (1986) Ovary slice culture of Lilium formosanum
Wallace. Japan. J. Breed. 36:304Å`308. |
|
Kanoh, K., M. Hayashi and
Y. Serizawa (1988) Production of interspecific hybrids between Lilium
longiflorum and L. elegans by ovary slice culture. Japan
J. Breed. 38:278Å`282. |
|
Kasumi, M., Y. Takatsu,
T. Manabe and M. Hayashi (2001) The effects of irradiating gladiolus
(Gladiolus x grandiflora hort.) cormels with gamma rays on
callus formation, somatic embryogenesis and flower color variations
in the regenerated plants. J. Japan Soc. Hort. Sci. 70:126-128. |
|
Kunishige, M and Hirata
Y. (1978) Studies on crosses of lilies: on the mixed pollen method.
Jpn bull Hortic. Res. Sta. 47:80-85. |
|
McRae, J. F. (1987) Stomata
size and pollen characteristics as an indication of chromosome numbers
in lilies. Lily yearbook, N. Am. Lily Soc. 40, 19-26. |
|
Myodo, H. (1942) Effects
of X-rays upon tulip plants when irradiated in different developmental
stages of floral organs. J. Fac. Agric. Hokkaido Imp. Univ., 48: 359-382. |
|
Nakajima, Y. (1940) On
the utility of the stored Lilium pollen and abnormal seed in crosses
of L. speciosum x L. auratum, L. speciosum x
L. makinoi. Bot. Mag. Tokyo 54:473-483. |
|
Nagatomi, S., A. Tanaka,
A. Kato, H. Yamaguchi, H. Watanabe and S. Tano (1998) Mutation induction
through ion beam irradiation in rice and chrysanthemum. TIARA Ann.
Rep. 7:41-43. |
|
Nezu, M. (1962) The effect
of radiation on tulip breeding. Gamma Field Symp , 1 : 43-49.
Nezu, M. (1963a) Studies on the production of bud sports in tulips
by ionizing radiation III. Frequency and mechanism of color formation.
Seiken Jiho, 15 : 75-86. |
|
Nezu, M. (1963b) Studies
on the production of bud sports in tulips by ionizing radiation IV.
Comparison of color sports by gamma-rays with similar virus disease
phenomena. Seiken Jjho, 15: 87-97 . |
|
Nezu, M. (1964) Studies
on the production of bud sports in tulips by ionizing radiation V.
Effects of total dose, fractionation and temperature on somatic mutation.
Jpn. J Genet., 39(6): 440-446. |
|
Nezu, M. (1965) Studies
on the production of bud sports in tulips by ionizing radiation VI.
Selection and observation of the mutant progeny. Jpn. J. Breed., 15(2):
113-11 8 |
|
Nezu, M. (1967) Tulip breeding
by bud sports induced by gamma rays. Toyama Agric. Exp. Stn, Spec.
Rep., No. 7, pp. 1-74 |
|
Nezu, M. and S. Obata (1964a)
Studies on the induction of bud sports in tulips by ionizing radiation.
I. Morphology and development of the bulbs. Jap. Jour. Genet, 38:386-391
. |
|
Nezu, M. and S. Obata (1964b)
Studies on the induction of bud sports in tulips by ionizing radiation.
II. Acute and chronic radiation effects on plant growth and bulbil
yield. Jap. Jour. Genet, 38: 392-398. |
|
North, C. and A.B.Wills
(1969) Inter-specific hybrids of Lilium lankongense Franchet
produced by embryo-culture. Euphytica 18:430-434. |
|
Okazaki, K., Y. Umada,
O. Urashima, J. Kawada, M. Kunishige and K. Murakami (1992) Interspecific
hybrids of Lilium longiflorum and L. x formolongi
with L. rubellum and L. japonicum through embryo culture.
J. Japan. Soc. Hort. Sci. 60:997-1002. |
|
Okazaki, K., Y. Asano and
K. Oosawa (1994) Interspecific hybrids between Lilium Oriental
Hybrid and L. Asiatic Hybrid produced by embryo culture with revised
media. Breed. Sci. 44:59-64. |
|
Okazaki, K. (1996) Lilium
species native to Japan, and breeding and production of Lilium
in Japan. Acta Hort. 414:81-92. |
|
Okazaki, K. and Y. Hane
(2002) Comparison between diploid and tetraploid forms of the same
clones of Lilium 'Asiatic Hybrid' under natural and early forcing
culture. (submitted for publication) |
|
Raven, P., R.F. Evert and
S.E. Eichhorn (1999) Biology of Plants. W.H.Freeman and Company, US. |
|
Ronald, W. G. and P. D.
Ascher (1976) Lilium x 'Black Beauty' - A potential bridging
hybrid in Lilium. Euphytica 25:285-291. |
|
Shibata, M. and J. Kawata
(1986) Chimerical structure of Marble sports series in chrysanthemum.
Development of new technology for identification and classification
of tree crops and ornamentals. Fruit Tree Research Station, MAFF.
Japan. 47-52. |
|
Shibata, M., S. Kishimoto,
M. Hirai and R. Aida (1998) Analysis of the periclinal chimeric structure
of chrysanthemum sports by random amplified polymorphic DNA. Acta
Hort. 454:347-353. |
|
Stettler R.F. (1968) Irradiated
mentor pollen: its use in remote hybridization of black cottonwood.
Nature 219:746-747. |
|
Tilney-Bassett, R.A.E.
(1986) Plant chimeras. Edward Arnold. |
|
Ureshino, K., I. Miyajima
and Y. Ozaki (2000) Overcoming for cross incompatibility between yellow-flowered
deciduous and evergreen azaleas with mentor pollination. J. Japan.
Soc. Hort. Sci. 69 (suppl.1):132. |
|
Van Harten, A.M (2002)
Mutation breeding of vegetatively propagated ornamentals.p.105-127.
In: A. Vainstein (ed.) Breeding for ornamentals: classical and molecular
approaches. Kluwer Academic Publishers |
|
Van Tuyl J.M., M.P. Van
Dien, M.G.M. Van Creij, T.C.M. Van Kleinwee, J. Franken and R.J. Bino
(1991) Application of in vitro pollination, ovary culture and embryo
rescue for overcoming incongruity barriers in interspecifc Lilium
crosses. Plant Science 74:115-126. |
|
Van Tuyl J.M, M.Claramarcucci
and. Visser (1982) Pollen and pollination experiments VII. The effect
of pollen treatment and application method on incompatibility and
incongruity in Lilium. Euphytica 31:613-619. |
|
Visser T. (1981) Pollen
and pollination experiments IV. Mentor pollen and pioneer pollen techniques
regarding incompatibility and incongruity in apple and pear. Euphytica
31:305-312 |
|
|
page top↑ |